Copy link
Physiologic Changes at High Altitude
Last updated: 10/29/2024
Key Points
- Acclimatization is the body’s process of adapting to the low-oxygen environment of high altitudes.
- Physiologic changes at high altitude enhance oxygen delivery to tissues through respiratory, hematological, and cardiovascular adaptations.
- High altitude exposure increases the risk for acute mountain sickness, high-altitude pulmonary edema, and high-altitude cerebral edema.
Introduction to Acclimatization
- Acclimatization is the body’s process of adapting to the low-oxygen environment of high altitudes, ensuring sufficient oxygen delivery despite a reduced partial pressure of oxygen (PO2).1
- Physiological changes begin at intermediate altitudes (1500-2500 m) and become more pronounced at higher elevations (>2500 m). At extreme altitudes (>5800 m), severe hypoxemia and potential deterioration can occur despite proper acclimatization.1
- As altitude increases, barometric pressure decreases nonlinearly, and the PO2 at altitude is significantly reduced. This is despite the percentage of atmospheric oxygen remaining constant at approximately 21% (Figure 1).1,2
- This reduction in barometric pressure lowers alveolar oxygen pressure (PAO2), leading to less oxygen available for diffusion into the bloodstream and raising the risk of severe hypoxemia.1
- For example, barometric pressure decreases from about 760 mmHg (101 kPa) at sea level to around 380 mmHg (51 kPa) at extreme altitudes (5500 m) and approximately 250 mmHg (33 kPa) at the summit of Mount Everest (8848 m).2
- Without adequate acclimatization, severe hypoxemia at extreme altitudes can lead to loss of consciousness within minutes.2
- The body’s acclimatization mechanisms enhance oxygen delivery to tissues through respiratory, hematological, and cardiovascular adaptations, allowing individuals to perform strenuous activities even at altitudes above 8000 m.2
Respiratory Adaptations
- Upon exposure to high altitude, the body increases ventilation to raise PAO2 and enhances oxygen uptake through increased breathing rate and depth.1-3
- The respiratory drive is primarily influenced by chemoreceptors that detect changes in oxygen (O2), carbon dioxide (CO2), and hydrogen ion (H+) levels.3
- Central chemoreceptors in the medulla detect changes in H+ concentration in cerebral extracellular fluid. Increased H+ concentration stimulates breathing, while decreased levels inhibit it.3
- The blood-brain barrier prevents H+ from crossing; thus, increased CO2 concentration leads to greater carbonic acid production, which then dissociates into H+, thereby stimulating ventilation.3
- Peripheral chemoreceptors in the carotid sinus and aortic body respond to changes in O2, CO2, and H+ levels. These receptors stimulate ventilation when serum O2 concentrations decrease, a response known as the hypoxic ventilatory response.3
- Prolonged hyperventilation leads to a reduction in arterial CO2 levels (PaCO2), leading to a respiratory alkalosis.1-3
- The body adapts by increasing bicarbonate excretion in the kidneys. This reduces inhibition on both central and peripheral chemoreceptors, allowing continued increased ventilation.2,3
- As hyperventilation reduces PaCO2, PAO2 increases, partially offsetting the effects of a decreased barometric pressure.1-3
- The relationship between PAO2, PiO2 (inspired partial pressure of oxygen), and PaCO2 can be predicted using the alveolar gas equation:
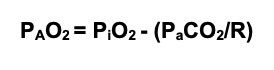
- Limitations in gas diffusion affect oxygen uptake in red blood cells as they pass through the alveolar-capillary interface because of reduced transit time from increased cardiac output and the high affinity of hemoglobin for oxygen (Figure 2).3
- Consequently, pulmonary diffusion becomes a major limiting factor for oxygen transport during aerobic exercise at high altitudes.3
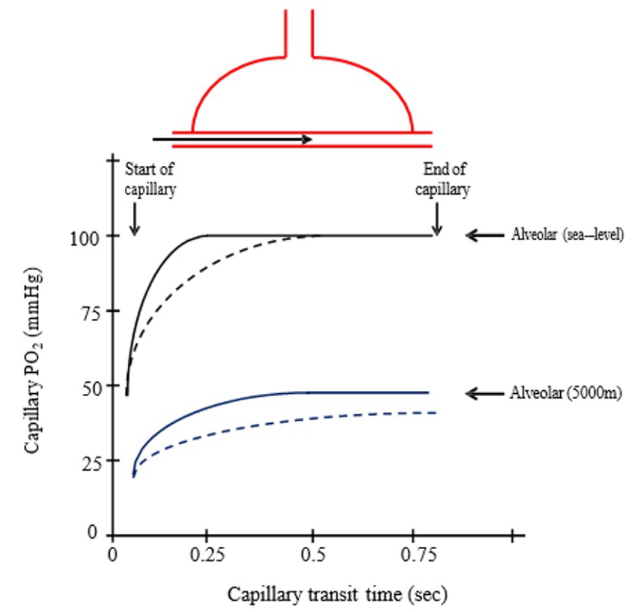
Figure 2. Changes in capillary PO2 vs. capillary transit time at rest (solid lines) and during exercise (dashed lines) at sea level (black lines) and 5000m (blue lines). Source: Ainsile P, Donnelly J. High Altitude Physiology- Climbing Earth’s Highest Peak. In: MacIntosh BR, Tschakovsky M, Fletcher JR. Open Textbook of Exercise Physiology. University of Calgary. Accessed October 23, 2024. CC BY-NC 4.0. Link
Cardiovascular Adaptations
- Acute altitude exposure causes an increase in heart rate, subsequently elevating cardiac output. This response is due to sympathetic nervous system activation triggered by hypoxic stimulation of peripheral chemoreceptors.1-3
- Chronic hypoxia gradually lowers heart rate; however, it remains above sea level values, resulting in a stabilized cardiac output above baseline. The increase in cardiac work is supported by coronary vasodilation and increased myocardial blood flow.1
- Stroke volume typically decreases with chronic altitude exposure; this reduction is primarily due to decreased end-diastolic volume resulting from a lower plasma volume rather than changes in myocardial contractility.3
- Hypoxic pulmonary vasoconstriction occurs when low oxygen levels lead to constriction of the pulmonary arterioles, shunting blood flow away from poorly ventilated regions of the lungs to better-ventilated areas and improving overall gas exchange.1-3
- This response leads to an increase in pulmonary artery pressure and pulmonary vascular resistance. In individuals with exaggerated hypoxic pulmonary vasoconstriction, there is a higher risk of developing high-altitude pulmonary edema and right ventricular strain.2,3
- Peripheral vasodilation occurs as an acute response to high altitude in a phenomenon known as “functional sympatholysis.” Chronic altitude exposure increases baseline sympathetic activity, leading to peripheral vasoconstriction and normalizing blood pressure.1,3
Hematological Adaptations
- Acute altitude exposure reduces plasma volume due to bicarbonate diuresis and fluid shifts, causing hemoconcentration. This increases hematocrit levels and improves oxygen delivery.1-3
- The kidneys respond to hypoxia by releasing erythropoietin, which stimulates the bone marrow to produce more red blood cells.2,3
- This response is mediated by the induction of the Hypoxia Inducible Factor (HIF-1α) gene, with erythropoietin levels peaking after approximately 48 hours of high-altitude exposure.2
- Elevated hemoglobin concentrations lead to increased blood viscosity and vascular resistance.3
- Chronic mountain sickness may develop in some individuals, characterized by extremely high hemoglobin levels (>22 g/dL) and symptoms such as headache, malaise, pulmonary hypertension, and cyanosis.3
- The oxygen-hemoglobin dissociation curve initially shifts to the left due to respiratory alkalosis and hypocapnia, enhancing hemoglobin’s affinity for oxygen and aiding oxygen uptake in the lungs (Figure 3).2,3
- With chronic altitude exposure, red blood cells increase production of 2,3-DPG, shifting the curve back to the right and promoting oxygen release at the tissues.2,3
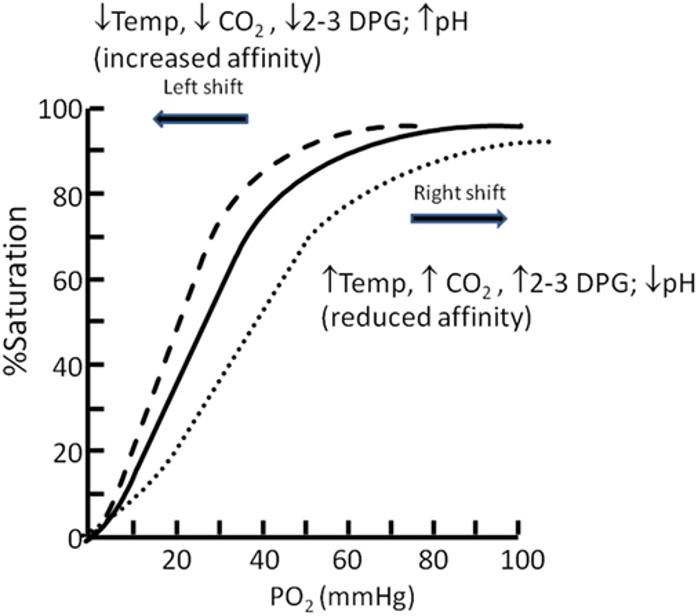
Figure 3. Oxygen dissociation curve. Source: Langley R, Cunningham S. Front Pediatr. 2017. CC BY. Link
Other Physiological Changes
- Cerebral vasodilation occurs during ascent to high altitudes, increasing cerebral blood flow by up to 20-30%. This response helps maintain adequate cerebral oxygen delivery, even at extreme altitudes.3
- The pathophysiology of acute mountain sickness and high-altitude cerebral edema is hypothesized to involve these processes of cerebral vasodilatation and microcirculatory changes, increasing capillary hydrostatic pressures and resulting in cerebral edema.2,3
- Chronic exposure to high altitude can cause weight loss and muscle atrophy due to dehydration, reduced energy intake, and increased basal metabolic rate.3
- Despite the increased basal metabolic rate, muscle tissue experiences a reduction in mitochondrial density, which decreases the efficiency of oxidative phosphorylation.2
- High-altitude exposure triggers hormonal changes that affect fluid balance and cardiovascular responses, including decreased renin-aldosterone activity, increased cortisol levels, and elevated catecholamines.4
- Long-term adaptations include increased baseline sympathetic activity and hypertrophy of the adrenal glands.4
Clinical Considerations at Altitude
- Exposure to high altitude increases the risk for various pathologies, including acute mountain sickness (AMS), high-altitude pulmonary edema (HAPE), and high-altitude cerebral edema (HACE).1-3
- Risk factors for developing these conditions include rapid ascent, insufficient acclimatization, and climbing to higher elevations.1,2
- Prevention strategies for altitude-related illnesses include gradual ascent, adequate hydration, limiting physical exertion, and potential use of medications such as acetazolamide or dexamethasone.1,2
- Asymptomatic individuals with no functional limitations typically do not require screening before ascending.1
- High altitude increases myocardial oxygen demand, which can increase the risk of ischemia in those with underlying cardiovascular disease. These individuals should avoid excessive exertion and consider using supplemental oxygen at high altitude.1
References
- Moulson N, Baggish A, Guseh JS. Exercise and Elevation. American College of Cardiology. Published March 15, 2021. Accessed October 13, 2024. Link
- Brown JP, Grocott MPW. Humans at altitude: physiology and pathophysiology. Continuing Education in Anaesthesia Critical Care & Pain. 2013;13(1):17-22 Link
- Ainsile P, Donnelly J. High Altitude Physiology- Climbing Earth’s Highest Peak. In: MacIntosh BR, Tschakovsky M, Fletcher JR. Open Textbook of Exercise Physiology. University of Calgary. Accessed October 23, 2024. Link
- Endocrine and Renal Function. In: Luks AM, Ainsile PN, Lawley JS, Roach RC, Simonson TS. High Altitude Medicine and Physiology. 6th ed. CRC Press; 2021: 281-308.
Copyright Information
This work is licensed under a Creative Commons Attribution-NonCommercial-NoDerivatives 4.0 International License.