Copy link
Pediatric Ventilation: Modes of Ventilation
Last updated: 04/18/2024
Key Points
- Modern anesthesia ventilators are well suited to ventilating pediatric patients in the operating room. Key advances in ventilator design that support pediatric ventilation include compliance compensation and eliminating the impact of fresh gas flow on tidal volume. These advances are reviewed in the OA summary entitled Pediatric Ventilation in the Operating Room – Equipment. Link
- Multiple ventilator modes are available to provide both controlled and supported spontaneous ventilation. Selecting the appropriate ventilator mode requires knowledge of the advantages and disadvantages of each mode to achieve the desired goals for gas exchange and lung-protective ventilation.
- Monitoring pressure and flow waveforms provide important insight into the interaction between the ventilator mode and the patient. Spirometry loops (pressure-volume and flow-volume) are available on many anesthesia machines and can help to optimize the ventilation strategy.
- If the goal is to assess oxygenation using a pulse oximeter, it is essential to reduce the fraction of inspired oxygen concentration (FiO2). The common practice of using enriched oxygen concentration can obscure oxygenation problems when using a pulse oximeter to assess oxygenation. In patients with normal lung function at baseline, typically the FiO2 should be 25% or less to detect impaired oxygenation.
Commonly Available Ventilation Modes
- Primary considerations in selecting a ventilator mode are the desire for a pressure or a volume target with each breath and whether or not spontaneous breathing efforts will occur. Controlled ventilation modes provide mandatory minute ventilation based on the ventilator settings. Supported ventilator modes are used when there is spontaneous breathing but do not guarantee a specific tidal volume or minute ventilation. Modern anesthesia ventilators offer both controlled and supported modes and the ability to mix controlled and supported modes similar to synchronized intermittent mandatory ventilation used in intensive care unit (ICU) ventilators.1,2
Basic Ventilator Modes
- In these modes, the ventilator function is independent of the patient’s lung compliance. The user sets a pressure or volume target and the other key parameters, which include inspiratory time (i-time) and respiratory rate (RR) that will determine the I:E ratio. It is convenient to set the I:E ratio (typically 1:2) directly along with the RR, which will determine the i-time. There are some additional settings that are useful and unique to each of the ventilator modes. Common basic ventilator modes include:
- Volume-Controlled Ventilation (VCV) – Volume target is set and pressure varies with lung compliance. The user sets a volume target and the parameters that determine the i-time. The ventilator divides the volume target by the i-time to determine the flow required to reach the volume target. The flow waveform is a square wave with a resulting pressure waveform that reaches peak inspiratory pressure at the end of inspiration (Figure 1A). Peak inspiratory pressure results from both flow through the resistance of the endotracheal tube plus the lung compliance. A pressure limit can be set to protect the lung from too much pressure, but the desired target volume will not be delivered if the pressure limit is reached. Setting an inspiratory pause causes the flow to stop before the end of inspiration and is useful to be able to assess plateau pressure. Plateau pressure is the best indicator of lung compliance since it is the pressure in the lung in the absence of flow (Figure 1B).
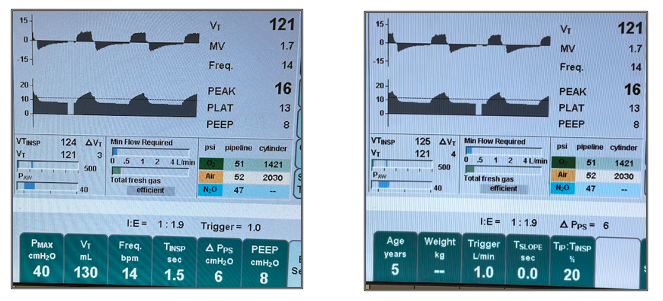
Fig 1A. Constant flow of 5.2 L/min from tidal volume divided by i-time (left); Fig 1B. Plateau pressure is evident when the inspiratory pause is set to 20% of i-time. Note that the flow stops but exhalation starts after the pause (right).
- Pressure-Controlled Ventilation (PCV) – Pressure target is set and volume varies with lung compliance. The user sets a pressure target and the parameters that determine the i-time. The ventilator will generate enough flow to reach the pressure target for the entire i-time, resulting in a square wave pressure waveform (Figure 2A). This pressure waveform favors lung recruitment since the maximum inspiratory pressure is present for the entire i-time. The flow waveform that results is a rapid rise to peak flow, which tapers or descends until exhalation begins (Figure 2B). Since the ventilator is controlled to maintain the target inspiratory pressure, as the lung fills, the lung compliance contributes to the target pressure, and less flow is required to achieve the pressure target.
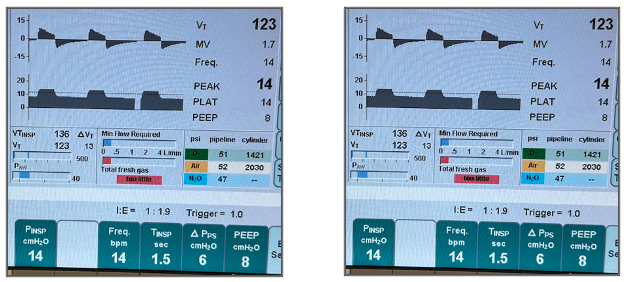
Fig 2A. Constant pressure for the entire i-time (left); Fig 2B. Initial maximum flow with tapering required to maintain a constant target pressure as the lung fills (right)
- While it may appear that one can achieve the same tidal volume with less pressure in PCV compared with VCV, this is not the case. For a given lung compliance, the pressure required to achieve a given tidal volume is the same for all ventilation modes. Peak pressure will typically be greater in volume mode due to the pressure generated by flow through the airway resistance, but the lungs are exposed to plateau pressure.
- Pressure Support Ventilation (PSV) – The user sets a pressure target or support pressure (Psup) that will be reached by the ventilator when the patient initiates a breath (Figure 3). The inspiratory pressure can be set as an absolute value or a pressure change or delta above positive end-expiratory pressure (PEEP). The resulting tidal volume is a combination of the patient’s effort and the Psup. Pressure support is very useful when a patient is breathing spontaneously to offset the work of breathing in the circuit, ensuring an adequate tidal volume especially when the effects of anesthetics or muscle relaxants impair the patient’s respiratory efforts. The flow threshold for triggering the ventilator to deliver a breath is typically adjustable. A low flow trigger is typically desirable in small patients but can result in false triggering if set too low. PEEP is typically set in addition to the inspiratory pressure. While the respiratory rate in PSV is typically set by the patient, it is possible to set a minimum rate to be delivered if the patient’s respiratory efforts become too low or stop completely. If the patient’s efforts cease, PSV will function like PCV and the measured respiratory rate will equal the minimum set rate. Note that pressure and flow waveforms in PSV are identical to PCV since they are both pressure-targeted modes of ventilation.
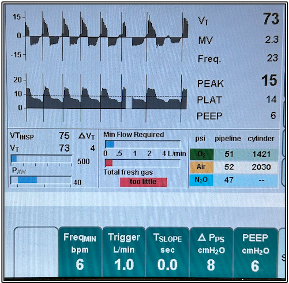
Figure 3. Pressure support ventilation. The patient is breathing at 23 bpm. The inspiratory pressure at 14 is PEEP plus the set inspiratory delta. The vertical spikes indicate triggered breaths. Other ventilators use different graphics to indicate triggered breaths.
- Continuous Positive Airway Pressure (CPAP): This mode is essentially a very limited type of PSV and is used when the patient is breathing spontaneously to offset the work of breathing imposed by the breathing circuit and artificial airway. The user sets the target pressure to be maintained constant during both inspiration and expiration. In essence, the Psup is equal to the PEEP value, although it is not set explicitly. There is typically no additional settings beyond the CPAP pressure setting, such as a backup respiratory rate or any trigger setting.
Advanced Ventilation Modes
- Not too long ago, anesthesia ventilators offered only controlled ventilation with volume or pressure targets. Pressure support ventilation was the first advance in anesthesia ventilators to support spontaneous ventilation. Currently, many anesthesia ventilators have incorporated ventilator modes that mimic, if not equal, the capabilities of ICU ventilators. Some examples include:
- Volume-targeted, pressure-controlled ventilation: This mode is intended to combine the best features of both VCV and PCV, i.e., a square wave pressure waveform with a volume guarantee. The user sets a volume target, and the ventilator determines the pressure needed for the set i-time to deliver the set tidal volume. The pressure waveform is a square wave and the resulting flow waveform is a descending pattern – identical to PCV. Determining the pressure required to generate the set tidal volume requires the ventilator to measure the patient’s lung compliance. To do so, the ventilator delivers a breath and measures the resulting tidal volume. After a few breaths, the ventilator will typically deliver the set tidal volume, and lung compliance is assessed with each breath to make adjustments as needed to ensure that the set tidal volume is delivered. While useful, this mode is less desirable when there are frequent changes in lung compliance (e.g., thoracoscopy), or when delays in reaching the desired tidal volume can result in lung derecruitment. There are a variety of names for this mode depending upon the manufacturer and all function similarly. Some examples include PCV-VG (GE Healthcare), Autoflow (Draeger Medical), PRVC (Mindray) and PRVC (Getinge).
- Airway Pressure Release Ventilation (APRV): This mode of ventilation has been a feature of ICU ventilators for many years and was developed to maintain lung recruitment in patients with significant lung injury and support spontaneous ventilation. It is not commonly used but is available on some anesthesia ventilators. Therefore, APRV will not be discussed in detail. It can be useful to continue APRV in the OR if it is available and successfully used when the patient is in the ICU.
Pros and Cons of Ventilation Modes
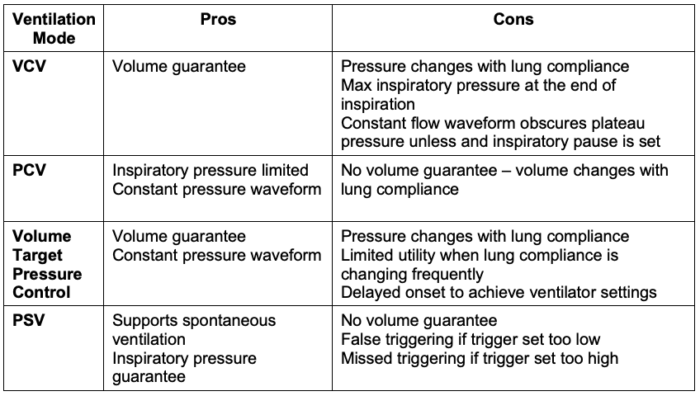
Table 1. Pros and cons of different ventilation modes
Monitoring Ventilation – Gas Exchange and Lung Compliance
- Monitoring ventilation on a breath-to-breath basis is essential to ensuring patient safety. A dislodged airway or unintended cessation of mechanical ventilation can be readily detected with appropriate patient monitoring. Monitoring is also useful for identifying gas exchange problems and optimizing the ventilation strategy. Overall, the goals are to achieve the best oxygenation at the lowest FiO2, acceptable carbon dioxide (CO2) elimination, and the desired tidal volume at the least pressure. Arterial blood gas analysis is the gold standard for assessing gas exchange but is not readily available for most patients. More common, noninvasive methods for assessing gas exchange will be the focus here.
- Gas Exchange – Estimating oxygenation using pulse oximetry3
- Pulse oximetry is the most common monitor used to assess oxygenation.
- While extremely valuable as a monitoring modality, pulse oximetry estimates only the saturation of hemoglobin, not the partial pressure of oxygen in the blood. As such, the pulse oximeter will reach the maximum saturation of 100% for PaO2 values greater than 95 mmHg.
- Minimizing the FiO2 is essential for using pulse oximetry effectively to assess oxygenation. In general, the FiO2 should be 0.25 or less for the pulse oximeter to be sensitive for detecting impaired oxygenation. If there is a potential risk to continuously reduced FiO2, the FiO2 should be reduced periodically to see if therapeutic efforts, such as specific ventilator settings or a recruitment maneuvers, are necessary and successful when executed.
- Gas Exchange – Ventilation (CO2 elimination)
- Tidal volume measurement: Measuring tidal volume is fundamental to assessing if the ventilator is functioning as intended. Tidal volume measurement is standard on virtually all anesthesia machines and is derived from a flow sensor. Basic machines have a single sensor at the expiratory valve. More advanced machines provide an additional sensor at the inspiratory valve. Tidal volume monitoring that best estimates the volume delivered to the patient requires an anesthesia machine with compliance compensation. When inspiratory and expiratory flow sensors are present, tidal volume measurements will not agree exactly. They should be within about 10% of the intended tidal volume; otherwise, a leak in the circuit is likely.
- Capnography: Measuring the inspired and expired concentration of CO2 is considered a monitoring standard during anesthesia and should always be available. Expired CO2 is typically measured as the end-tidal concentration (EtCO2) and is intended to be the best estimate of the partial pressure of arterial CO2 (PaCO2). There will always be a difference or gradient between ETCO2 and PaCO2 of at least 5 mmHg. An adequate tidal volume of at least 6 mL/kg and limiting the dead space added to the circuit are important to minimizing the gradient. That said, PaCO2 cannot be estimated with certainty from ETCO2, and arterial blood gas measurement is necessary when adequate CO2 elimination must be confirmed (e.g., intracranial pathology, pulmonary hypertension).4 Note: Volumetric capnography is another approach that is less commonly used and outside the scope of this discussion.
- Lung Compliance – Combining pressure and volume measurement5
- Lung compliance is determined from the ratio of tidal volume to inspiratory pressure and is calculated by many modern anesthesia machines from the measured values of those parameters. Lung compliance can be measured as either dynamic or static compliance. Reliable lung compliance assessment requires accurate measurement of the volume delivered to the patient’s lungs as well as inspiratory pressure measurement.
- Dynamic Lung Compliance: This is the ratio of tidal volume to peak inspiratory pressure. The term dynamic is used since this measurement can be done while there is flow in the breathing circuit. As such, it is not as useful an indicator of true lung compliance since it includes both the impact of compliance on pressure as the lung fills as well as the impact of pressure due to flow. Under stable conditions of flow and resistance, changes in dynamic compliance are useful trend indicators of changes in lung compliance.
- Static Lung Compliance: This is the ratio of tidal volume to inspiratory plateau pressure. By definition, the plateau pressure occurs when there is no flow in the breathing circuit. As such, it is the best indicator of intrinsic lung compliance. Static lung compliance can be assessed during VCV by setting an inspiratory pause typically at least 20% of the i-time. During PCV, if flow approaches zero during inspiration, calculated lung compliance will be close to static compliance.
- Spirometry is the breath-to-breath measurement of pressure and flow displayed as pressure-volume (PV) and flow-volume (FV) loops. Modern anesthesia machines that incorporate compliance compensation are able to measure volumes delivered at the airway using flow sensors within the anesthesia machine. These machines typically offer the ability to monitor PV and FV loops. PV loops are breath-to-breath indicators of lung compliance and are useful for optimizing mechanical ventilation. Most spirometry displays allow a reference or baseline loop to be saved so that changes in lung compliance will be easily visualized relative to the saved reference.
- PV loops need to be interpreted in the context of the ventilation mode being used. In particular, pressure-targeted and volume-targeted modes of ventilation will create different changes in the loops as lung compliance changes (Figures 4A and 4B).
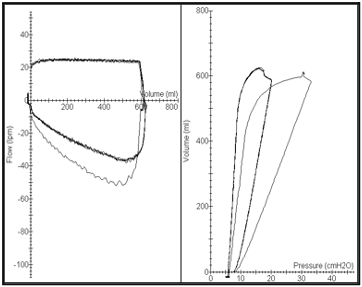
Fig 4A. VCV mode P/V Loop (right). Constant volume. Loop will move to the right if lung compliance worsens due to increased pressure.
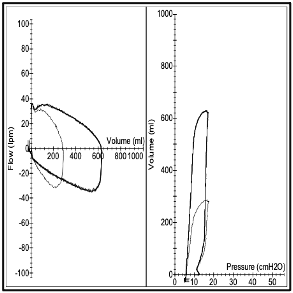
Fig 4B. PCV mode P/V Loop (right). Constant Pressure. Loop will decrease in height as lung compliance changes due to reduced volume.
References
- Feldman JM. Optimal ventilation of the anesthetized pediatric patient. Anesth Analg. 2015;120(1):165-75. PubMed
- Spaeth J, Schumann S, Humphreys S. Understanding pediatric ventilation in the operative setting. Part II: Setting Perioperative Ventilation. Pediatr Anaesth. 2022;32:247-54. PubMed
- Tusman G, Bohm SH, Suarez-Sipmann. Advanced uses for pulse oximetry for monitoring mechanically ventilated patients. Anesth Analg. 2017;124:62-71. PubMed
- Humphreys S, Schibler A, von Ungern-Sternberg BS. Carbon dioxide monitoring in children – A narrative review of physiology, value, and pitfalls in clinical practice. Paediatr Anaesth. 2021;31(8):839-45. PubMed
- Spaeth J, Schumann S, Humphreys S. Understanding pediatric ventilation in the operative setting. Part I: Physical principles of monitoring in the modern anesthesia workstation. Pediatr Anaesth. 2022;32:237-46. PubMed
Copyright Information
This work is licensed under a Creative Commons Attribution-NonCommercial-NoDerivatives 4.0 International License.