Copy link
Pediatric Ventilation: Breathing Circuits
Last updated: 04/30/2024
Key Points
- Different types of breathing circuits are available to anesthesia providers caring for pediatric patients in the operating room (OR) and intensive care units (ICU).
- Manual circuits are of two basic types -- self-inflating and Mapleson-type circuits -- and are typically used for transport, resuscitation, or backup when mechanical ventilators fail. Mapleson-type circuits are preferred.
- The modern circle anesthesia system is highly effective for pediatric patients due to features of compliance and fresh gas compensation, but a proper pre-use leak and compliance test is essential.
- While effective for mechanical ventilation, ICU ventilators are limited for anesthesia care by their inability to deliver inhaled anesthetics and easily transition to manual ventilation. Compact ICU ventilators are readily available and better than manual circuits for patient transport.
Manual Circuits: Self-Inflating and Mapleson Type
- Manual circuits are simple yet powerful tools in pediatric anesthesia that enable controlled ventilation by mask or artificial airway in virtually any environment. They are typically used for transport, resuscitation, and as a backup when a mechanical ventilator fails. There are two types: self-inflating and flow-inflating. Clinical use of these devices is discussed more extensively in the OA Summary entitled Pediatric Bag-Mask Ventilation.
- Self-inflating bags are sometimes known as Ambu bags in recognition of the original device developed by Ambu Corporation, but there are many manufacturers (Figure 1).
- The primary advantage of this design is its ability to provide positive pressure ventilation without an external gas supply, although it is better used with an oxygen supply.
- Unless the oxygen supply includes a blender and air source, only 100% oxygen is delivered.
- They are a useful design for low-resource settings and patient transport.
- They do not support blow-by oxygen for patients breathing spontaneously nor do they provide particularly good tactile feedback of lung compliance and tidal volume to the clinician while ventilating.
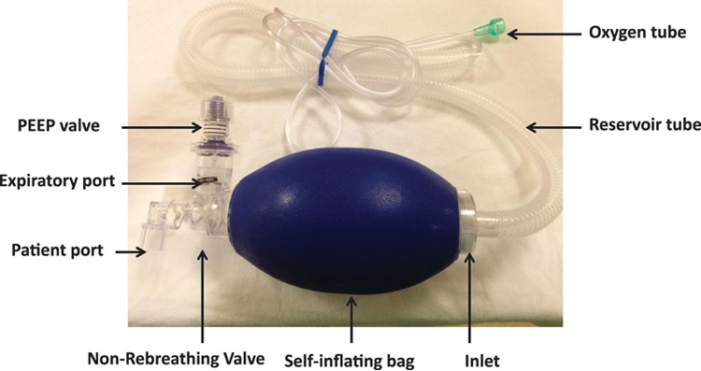
Figure 1. Self-inflating bag. Used with permission from Sivco CS, et al. A A Pract. 2018;11:288-91. PubMed
- Flow-inflating bags are most commonly the Mapleson-type design.
- They provide an excellent tactile feel of lung compliance and tidal volume.
- It can be easily used when patients are breathing spontaneously.
- Classically, they are described as types A-F based on the relative position of the gas inflow, exhalation path, and reservoir bag. The Mapleson D design has become the most popular due to its efficiency in eliminating carbon dioxide (CO2) (Figure 2).
- Other important caveats to using these circuits effectively include:
- Total fresh gas flow must be carefully controlled to avoid rebreathing exhaled CO2.
- Excessive fresh gas flow will reduce the tactile feel.
- Exhalation valves are typically manually adjustable and require expertise to ensure desired inspiratory pressure and positive end-expiratory pressure (PEEP), if needed.
- Simple manometers are often incorporated into the circuit and are useful adjuncts for adjusting the exhalation valve properly.
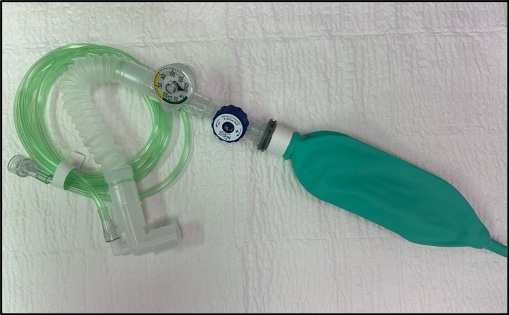
Figure 2. Flow Inflating bag
Circle Anesthesia System
- The circle anesthesia system has become the universal design for delivering inhaled anesthetics and positive pressure ventilation. While there are differences in the circle system design between anesthesia workstations related to the ventilator technology employed, the basic functionality of the circle system is the same (Figure 3).
- Details of the differences in some commonly available anesthesia ventilators and circle systems are described in the OA Summary entitled Pediatric Ventilation in the Operating Room: Equipment.
- The primary purpose of the circle anesthesia system is to provide a path for exhaled anesthetic gases to return to the patient rather than be wasted via the scavenging system. Whether or not exhaled gases are “rebreathed” depends upon how fresh gas flow is managed. The clinician who understands how to manage fresh gas flow effectively (aka low-flow anesthesia) can minimize the waste and pollution associated with inhaled anesthetic delivery.1
- The principles and practice of low-flow anesthesia are beyond the scope of this summary but are available from other sources including a course on low-flow anesthesia for anesthesia professionals developed by the Anesthesia Patient Safety Foundation.
- The basic components of the circle system are:
- Inspiratory and expiratory limbs with valves to ensure unidirectional flow
- Connection between the two limbs for exhaled gases to return to the patient
- Carbon dioxide absorbent to remove exhaled CO2 before it returns to the patient
- Source of fresh gas
- Anesthetic vaporizer
- Scavenging system
- Ventilation capabilities for manual and mechanical ventilation
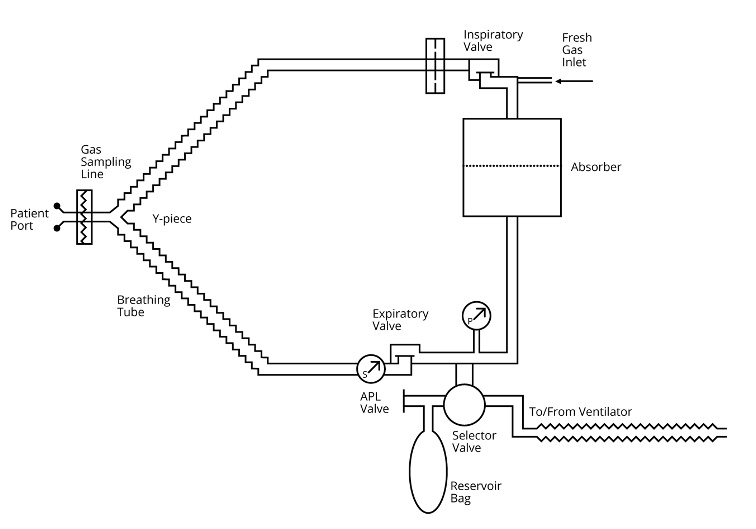
Figure 3. Basic circle system design
Using the Circle System to Deliver Inhaled Anesthetics
- Fresh gas flow settings are fundamental to providing safe and effective concentrations of gases (oxygen, nitrogen, nitrous oxide) and anesthetics in the circuit.
- Total fresh gas flow will determine both the rate at which circuit concentrations change and the amount of inhaled anesthetic wasted. While greater fresh gas flow provides the convenience of more rapid control over gas and anesthetic concentrations, it also contributes to more waste. Learning to manage the compromise between convenience and waste is the art of using the circle system. Continuous monitoring of gas and anesthetic concentrations is essential to safely and effectively managing fresh gas flow. Some workstation designs provide technological conveniences to make the process easier.2
- Generally, when total fresh gas flow equals minute ventilation, there is little to no rebreathing of exhaled gas. Consequently, fresh gas flow settings beyond that limit create waste without speeding induction. In pediatric patients, minute ventilation varies considerably based on patient size which creates an opportunity to limit inhaled anesthetic waste, especially during inhalation induction. A one-pager on weight-based settings for maximum fresh gas flow during inhalation induction has been published on the Society for Pediatric Anesthesia website.3 There is no clinical benefit to increasing fresh gas flow beyond minute ventilation.
- When using sevoflurane, the recommendation for a minimum fresh gas flow is well recognized as obsolete.4 Although the drug labeling recommends a minimum fresh gas flow of 2 L/min or 1 L/min for up to 2 MAC-hours, the literature clearly indicates that there is no risk of compound A toxicity in humans, and modern carbon dioxide absorbents do not produce compound A. Further, the American Society of Anesthesiologists has published a statement indicating that reduced fresh gas flow, even less than 1 L/min, is safe when using sevoflurane.4
Using the Circle System for Mechanical Ventilation
- Modern anesthesia ventilators are highly capable of ventilating even the smallest patients. These ventilators have specifications that match those of most ICU ventilators. Detailed information on anesthesia ventilator design and function is available from other sources. A few key points to keep in mind:
- Compliance compensation is an essential feature of modern anesthesia ventilators that makes them well-suited to ventilate even the smallest pediatric patients.5
- The pre-use leak and compliance test involves occluding the end of the breathing circuit so the ventilator can measure the circuit compliance. It is essential that once the compliance of the circuit is measured, nothing should be changed that would alter the circuit compliance, e.g., lengthening the circuit.
- Once the circuit compliance is measured, the ventilator will compensate for the compliance during volume-targeted ventilation to deliver the set tidal volume to the patient’s airway.
- Respiratory monitoring is also enhanced by compliance compensation in all modes of ventilation. Tidal volume measured by sensors remote from the airway is corrected to reflect the volume delivered to the airway. Spirometry (pressure-volume and flow-volume loops) is also possible.
- Fresh gas flow independence is another important feature of the modern anesthesia workstation, ensuring that tidal volume is unaffected by changes in fresh gas flow.
- The circle system offers the convenience of easy transitions between manual and mechanical ventilation, which is often needed when anesthetizing pediatric patients.
ICU Ventilators
- The basic design of an ICU ventilator is simple (Figure 4). There are pressurized sources of air and oxygen connected to the ventilator, a blender to provide the desired inspired oxygen concentration, and valveless inspiratory and expiratory limbs of the breathing circuit. The complexity of these ventilators is in the control circuits that deliver the desired ventilation pattern and the pressure and flow sensors that are essential to proper function.
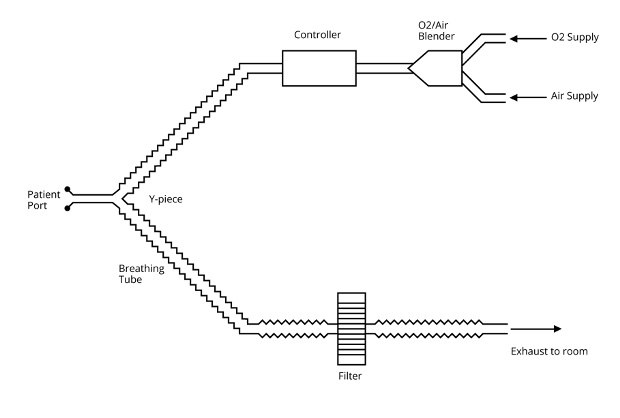
Figure 4. Basic ICU ventilator circuit design
- Since the pressurized supply gases become the inspired gases, there is no limit to the gas available to ventilate the patient. Anesthesia machines are fundamentally different since they separate the patient’s gas from the driving pressure. As a result, the volume available in the patient gas portion of the circuit (bellows, piston, turbine, volume reflector) limits the total volume of gas available to create a breath. Note: The volume reflector is a special case, but the details are beyond the scope of this discussion.
- This brief review is not intended to explore the ICU ventilator in detail but rather to highlight its role in anesthesia practice.
- Transporting Patients to and from the OR: A common approach when positive pressure ventilation is required during transport is to use a manual circuit, as described earlier. Manual circuits are not ideal since it is difficult to provide consistent inspiratory pressure, tidal volume, and PEEP, and typically, only 100% oxygen can be delivered. Fortunately, compact transport ventilators have become available in recent years that are capable of maintaining ICU-quality ventilation during transport. These ventilators are designed to work with compressed gas cylinders and have an internal battery that can power the ventilator for extended periods of time. While all patients requiring transport will benefit from using a transport ventilator, patients with underlying lung disease (e.g., acute respiratory distress syndrome) and abnormal gas exchange will certainly benefit from using a ventilator during transport.
- Use in the OR: When caring for a patient with significant lung disease in the OR, it can be challenging to provide positive pressure ventilation to achieve desirable oxygenation and ventilation. In these cases, an ICU ventilator is sometimes considered an alternative to the anesthesia ventilator. Given the capabilities of modern anesthesia ventilators, it is rarely necessary to use an ICU ventilator to provide effective positive pressure ventilation, even for patients with major pulmonary impairment. While a high-quality ICU ventilator is certainly the gold standard for mechanical ventilation, there are advantages to using an anesthesia ventilator in the OR.
- Advantages of using an anesthesia ventilator compared with an ICU ventilator in the OR
- Delivery of inhaled anesthetics
- Easy transition between manual and mechanical ventilation without interrupting ventilation
- Already present in the OR
- Disadvantages of using an ICU Ventilator in the OR
- To transition between manual and mechanical ventilation, the ventilator circuit must be disconnected from the airway and a manual ventilation device connected. This process interrupts ventilation, often leading to de-recruitment of alveoli, and requires manipulating the airway under the surgical drapes.
- Crowding in the OR due to an extra piece of equipment that requires connection to gas supplies and placement close to the patient’s airway.
- Anesthesia providers may be unfamiliar with the controls required to optimize ventilator function as needed.
- While modern anesthesia ventilators mimic the capabilities of ICU ventilators, achieving the best performance in pediatric patients requires careful attention to ensuring a proper leak and compliance test is performed with all of the circuit devices in place. No change to circuit compliance should be made (e.g., extending a circuit length) after the test is done. Dead space in the breathing circuit must be optimized to ensure it is no more than 30% of the intended tidal volume.
- One more comment about minimum tidal volume specifications in ICU and anesthesia ventilators — ICU ventilators designed for use with small infants will have a minimum tidal volume specification that is lower than anesthesia ventilators. Achieving performance at that minimum specification requires a flow sensor mounted between the anesthesia circuit and the patient’s airway. Anesthesia ventilators are not designed to function with an additional flow sensor in the airway.
References
- Feldman JM. Managing fresh gas flow to reduce environmental contamination. Anesth Analg. 2012;114(5):1093–1101. PubMed
- Feldman JM, Hendrickx J, Kennedy RR. Technology and Low-Flow Anesthesia Practice. Accessed April 9, 2024. Link
- Glenski T, Narayanasamy S. Low-flow anesthesia in pediatric patients. SPA One-Pager. 2022. Accessed April 9, 2024. Link
- Statement on the Use of Low Gas Flows for Sevoflurane. ASA Committee on Equipment and Facilities. 2023. Accessed April 9, 2024. Link
- Feldman, JM. Optimal ventilation of the anesthetized pediatric patient. Anesth Analg. 2015;120(1):165–75. PubMed
Other References
- Mapleson breathing systems. In: Understanding Anesthesia Equipment. Dorsch JA, Dorsch SE (eds). 5th edition. 2008. Lippincott Williams and Wilkins, Philadelphia, PA.
- Additional information is available in an OA Summary on Anesthesia Breathing Systems. Link
Copyright Information
This work is licensed under a Creative Commons Attribution-NonCommercial-NoDerivatives 4.0 International License.