Copy link
Effects of Anesthesia on the Respiratory System
Last updated: 04/09/2025
Key Points
- In the anesthetized patient, respiratory compliance decreases, resistance increases, and FRC decreases, which may create a ventilation-perfusion mismatch.
- Atelectasis is commonly observed in anesthetized patients secondary to airway closure promoted by decreased functional residual capacity and a high fraction of inspired oxygen. It may persist for days in the postoperative period and is a source of pulmonary complications.
- Ventilation and perfusion abnormalities may be observed in the anesthetized patient, including increased dead space, intrapulmonary shunt, impaired hypoxic pulmonary vasoconstriction, and increased dispersion of ventilation to perfusion ratios.
Effects of Anesthesia on Lung Mechanics
- There is a decrease in functional residual capacity (FRC) during the induction of anesthesia, which is attributable to patient positioning and loss of muscle tone.1,2
- Change in position from upright to supine results in a reduction in FRC by 0.8-1.0 L.1
- With the induction of general anesthesia, there is an additional decrease in FRC by 0.4-0.5 L, even as spontaneous breathing is maintained.1
- Muscle paralysis or mechanical ventilation have not been shown to decrease FRC further.1
- The loss of inspiratory muscle tone, particularly the cranial displacement of the diagram, appears to be the primary reason for the decrease in FRC with induction.1
- Induction of anesthesia with ketamine is a notable exception to the above pattern. As muscle tone is preserved during ketamine induction, the typical reduction in FRC with induction is not observed.1
- FRC may be further reduced by abdominal insufflation in laparoscopic procedures and/or Trendelenburg position, which increases abdominal pressure, limiting diaphragmatic excursion.2
- The decreased FRC following induction of anesthesia does not vary with anesthetic depth and may take hours to days to normalize following emergence.2
- The decrease in FRC during anesthesia may cause FRC to exceed closing capacity, promoting airway closure, particularly in dependent regions of the lungs, resulting in an intrapulmonary shunt.1
- There is a biphasic relationship between airway closure and patient age. The likelihood of airway closure decreases to an age of approximately 20 years and increases after that. This may be explained by the small airways being optimally supported as the lungs reach maturity and declining as loss of elasticity increases the propensity for airway collapse.1
- Positive airway pressure, whether delivered by application of positive end-expiratory pressure (PEEP) or continuous positive airway pressure (CPAP), increases transpulmonary distending pressure and can, therefore, increase FRC above closing capacity and may decrease intrapulmonary shunting.3
- The motion of the diaphragm is altered during passive motion in mechanical ventilation as opposed to when acting as a respiratory muscle.1
- During active inspiration, the dorsal portion of the diaphragm is typically displaced caudally to a greater degree than the ventral portions.1
- However, during mechanical ventilation, the ventral portion of the diaphragm will experience greater movement than the dorsal, dependent portions due to the increased hydrostatic pressure from the abdomen placed on these dependent portions.1
- This alteration in diaphragmatic motion may alter the distribution of ventilation within the lungs.1
- The static compliance of the respiratory system (including the lungs and chest wall) decreases by an average of 40 mL/cm H2O to an average value of 60 mL/cm H2O during anesthesia.1
- Resistance of both the lungs and total respiratory system is increased during anesthesia.1
- It is unclear whether this increase in resistance is attributable to factors beyond reduced FRC.1
- The increased resistance that would be expected with reduced FRC may be negated by the bronchodilatory properties of inhalational anesthetics.2
- Pathological factors (upper airway obstruction, laryngospasm, bronchospasm, blood, or secretions in the airway, etc.) and equipment issues (small or kinked endotracheal tube, kinked circuit, malfunctioning valves, etc.) can increase airway resistance.2
Anesthesia and Atelectasis
- Atelectasis may be seen in about 90% of all anesthetized patients. It is typically most prominent at the lung bases and decreases toward the apex. It is observed with both spontaneous and mechanical ventilation and during intravenous or inhaled anesthetics.1
- Mechanisms of atelectasis in the anesthetized patient include absorption atelectasis, compression atelectasis, and surfactant deficiency.
- In absorption atelectasis, gas is resorbed in a region with a low ventilation-to-perfusion ratio (V/Q), such as alveoli distal to an obstruction, leading to alveolar collapse. This form of atelectasis develops most quickly in the presence of highly soluble gas mixtures such as 100% oxygen.3,4
- In compressive atelectasis, dependent regions of the lung may collapse under the weight of the chest wall and abdomen. This has not been proven to be as significant of a contribution to atelectasis during anesthesia as absorption atelectasis.1,4
- Surfactant production may decrease during general anesthesia, and a lack of deep breaths in the mechanically ventilated patient may result in decreased active surfactant, leading to further alveolar collapse. This mechanism of atelectasis is thought to be more prominent in the setting of prolonged mechanical ventilation.4,5
- 10-20% of the lung area may be atelectatic after the induction of anesthesia before the surgical procedure has started.1
- The incidence of atelectasis formation during anesthesia does not appear to increase with age.1
- The extent of atelectasis varies by the surgical procedure
- In thoracic surgical procedures and cardiopulmonary bypass, over 50% of the lungs may be atelectatic several hours after the procedure.1
- Abdominal surgery does not appear to contribute significantly to atelectasis.1
- Pneumoperitoneum in laparoscopic procedures contributes to greater atelectasis; however, arterial oxygenation seems to be improved during carbon dioxide pneumoperitoneum, possibly due to enhanced hypoxic pulmonary vasoconstriction due to the CO2.1
- Mechanisms of atelectasis in the anesthetized patient include absorption atelectasis, compression atelectasis, and surfactant deficiency.
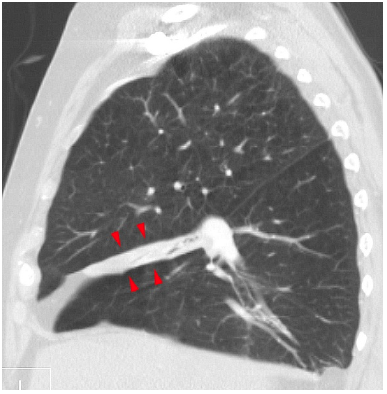
Figure 1. Right middle lobe atelectasis visualized on sagittal CT scan. Source: Hellerhoff at Wikimedia Commons, CC BY-SA 3.0 Link
- Multiple strategies may be employed to prevent or reverse atelectasis during anesthesia, including recruitment maneuvers and PEEP.
- Closure of airways is the most significant contribution to atelectasis during anesthesia. Increased oxygen concentration corresponds with faster gas resorption and atelectasis formation.1
- The use of a PEEP of 6 cm H2O during anesthesia in a patient with a body mass index (BMI) of less than 25 may reopen collapsed airways and, if applied early, may prevent the formation of atelectasis.1
- Increased PEEP may also reopen previously collapsed alveoli, though an improvement in arterial oxygenation may not be observed due to blood flow shift to additional dependent, atelectatic regions and deranged hemodynamics.1
- Sustained inflation of the lungs (recruitment maneuver) at 30 cm H2O decreases the amount of atelectasis by about half, while an airway pressure of 40 cm H2O is required in a healthy weight anesthetized patient to reopen all collapsed alveoli. These pressures are increased in patients with higher BMI.1
- Changes in the fraction of inspired oxygen (FiO2) utilized in different phases of an anesthetic case contribute to changes in atelectasis.
- Preoxygenation before induction of anesthesia with 100% oxygen is a standard practice to prolong apnea tolerance time. However, the high FiO2 present at this time also contributes to the formation of atelectasis, which may itself decrease the apnea tolerance time.1
- Ventilating with 30% rather than preoxygenating with 100% oxygen at induction of anesthesia appears to prevent atelectasis at induction, and when patients were examined for atelectasis at differing fractions of inspired oxygen at induction, atelectasis was seen in all patients at 100% oxygen, but in progressively fewer patients preoxygenated with 80% and 60% oxygen.1
- CPAP at induction has been proposed as a method to prevent the decreased FRC and atelectasis observed at induction while also allowing preoxygenation with high FiO2. However, questions remain regarding the potential for stomach insufflation and subsequent aspiration risk.1
- During the maintenance phase of anesthesia, moderate FiO2 and PEEP following a recruitment maneuver may be utilized to avoid the rapid atelectasis reformation that may occur at high FiO2 levels.1
- During emergence from anesthesia, high FiO2 is again typically utilized to decrease hypoxemia risk, likely contributing to atelectasis. Proposed strategies to reduce atelectasis during emergence include a recruitment maneuver and subsequent ventilation with lower FiO2 and the use of PEEP until extubation, followed by a limited duration of postextubation CPAP.1
- Preoxygenation before induction of anesthesia with 100% oxygen is a standard practice to prolong apnea tolerance time. However, the high FiO2 present at this time also contributes to the formation of atelectasis, which may itself decrease the apnea tolerance time.1
- Atelectasis may persist in the postoperative period for two days or more. Atelectasis is a likely locus for infection and a source of postoperative pulmonary complications.1,6
Effects of Anesthesia on Pulmonary Ventilation and Perfusion
- During anesthesia with mechanical ventilation, ventilation exceeds perfusion in the upper half of the lungs, giving the appearance of increased dead space, which impedes oxygenation and carbon dioxide elimination.1
- There is a significant alteration in pulmonary ventilation, while pulmonary perfusion typically does not vary dramatically from the awake state.6
- In the middle region of the lung, ventilation is reduced due to intermittent airway closure (low V/Q).6
- In the lower lung regions, ventilation may be absent due to further airway closure and subsequent atelectasis. If perfusion persists in this region, a shunt may be created.6
- Both the low V/Q in the middle lung and the shunt in the lower lung contribute to impaired oxygenation. High FiO2 will alleviate the effect of low V/Q but will worsen atelectasis and shunt. PEEP and recruitment maneuvers may increase ventilation of dependent portions of the lung.1,6
- In an anesthetized, mechanically ventilated patient, there is an increase in perfusion from the ventral to dorsal regions of the lungs.1
- There is some reduction in blood flow at the most ventral, atelectatic regions. Despite its utility in reducing atelectasis, the use of PEEP redistributes blood flow to the dependent lung regions, which may increase intrapulmonary shunt.1
- Higher levels of PEEP also impede right heart venous return and cardiac output.1
- Hypoxic pulmonary vasoconstriction (HPV) is the contraction of vascular smooth muscle in the pulmonary circulation in response to low local partial pressure of oxygen.7
- Modern volatile anesthetic agents, including halothane, sevoflurane, isoflurane, and desflurane, all inhibit HPV in a dose-dependent fashion. Of the volatile agents, halothane appears to be the most potent inhibitor of HPV, while the latter three agents appear to inhibit HPV equally for equivalent minimum alveolar concentration doses.7
- Commonly used intravenous anesthetics do not inhibit HPV, including propofol, despite its systemic vasodilatory properties.7
- The effect of nitrous oxide on HPV is not clear: though some animal studies have suggested it may inhibit HPV, this has not been observed in humans.7
- Anesthesia increases the dispersion of V/Q ratios in the lungs, reflecting uneven distribution of ventilation in relation to blood flow.1
References
- Hedenstierna G, Edmark L. Effects of anesthesia on the respiratory system. Best Pract Res Clin Anaesthesiol. 2015;29(3):273-84. PubMed
- Butterworth JF, Mackey DC, Wasnick JD. eds. Chapter 23: Respiratory physiology & anesthesia. In: Morgan & Mikhail’s Clinical Anesthesiology. seventh edition. McGraw-Hill LLC; 2022.
- Butterworth JF, Mackey DC, Wasnick JD. eds. Chapter 58: Inhalation Therapy & Mechanical Ventilation in the PACU & ICU. In: Morgan & Mikhail’s Clinical Anesthesiology. seventh edition. McGraw-Hill LLC; 2022.
- Hedenstierna G, Edmark L. Mechanisms of atelectasis in the perioperative period. Best Pract Res Clin Anaesthesiol. 2010;24(2):157-69. PubMed
- Akça O, Podolsky A, Eisenhuber E, et al. Comparable postoperative pulmonary atelectasis in patients given 30% or 80% oxygen during and 2 Hours after colon resection. Anesthesiology. 1999;91(4):991-8. PubMed
- Hedenstierna G. Oxygen and anesthesia: what lung do we deliver to the post‐operative ward? Acta Anaesthesiol Scand. 2012;56(6):675-85. PubMed
- Lumb AB, Slinger P. Hypoxic pulmonary vasoconstriction: Physiology and anesthetic implications. Anesthesiology. 2015;122(4):932-46. PubMed
Copyright Information
This work is licensed under a Creative Commons Attribution-NonCommercial-NoDerivatives 4.0 International License.