Copy link
Clinical Alarms and Alarm Fatigue
Last updated: 10/02/2024
Key Points
- Alarms are built into medical monitors for patient safety, and they warn providers of potentially life-threatening clinical changes.
- Healthcare providers may be exposed to hundreds of alarms daily, including false alarms that do not represent a true danger to patient safety.
- Alarm fatigue develops from excessive alarms and can cause a delay or lack of provider response, potentially leading to patient harm.
- Tailoring alarm thresholds to each individual patient and disabling nonessential alarms may help minimize alarm fatigue.
- Potential future solutions to alarm fatigue include changing alarm processing algorithms, developing multiparametric and/or trend-based alarms, and lowering alarm volume for nonessential alarms.
Introduction
- An alarm can be defined as “an unexpected change in system state, a means of signaling state changes, a means of attracting attention, a means of arousing the operator, and a change in the operator’s mental state.”1
- Medical devices, such as vital sign monitors and ventilator/anesthesia workstations, generate alarms to warn providers of significant physiologic changes or required actions.
- The current international standard for medical alarms is IEC 60601-8, which specifies basic safety and performance requirements for medical alarms. These standards specify which medical conditions should trigger an alarm, alarm frequency and volume, and how alarm patterns should vary based on urgency, among other technical specifications.2
Types of Alarms
- Anesthetic alarms can be broadly categorized into clinical and technical alarms.
- Clinical alarms indicate a change in the patient’s physiologic state.
- Technical alarms indicate an issue with the biomedical equipment itself (i.e., a disconnected sensor or an empty infusion pump).2
- Most alarms are threshold alarms, which trigger an acoustic and/or visual signal when a predefined threshold is violated (i.e., mean arterial pressure is above or below a predefined normal range).3
- Based on signal detection theory, given any reading from the environment, a monitor will determine whether the parameter is normal or abnormal (based on predefined decision criteria), and the resulting action can be classified into one of four categories (Figure 1):4
- Hit: a true alarm (i.e., there is an abnormal situation and the alarm sounds)
- Correct rejection: true normal (i.e., the situation is normal and there is no alarm)
- False alarm (i.e., the situation is normal, but the monitor detects an abnormality, and the alarm sounds)
- Miss (i.e., there is an abnormal situation that the monitor fails to detect, and there is no alarm)
- When a monitored parameter reaches a certain decision criterion, β, an alarm goes off. The effectiveness of the system is determined both by the stringency of the decision threshold as well as by how much overlap there is between “normal” and “abnormal” parameter distributions, which is referred to as the discriminability index, or d’ (Figure 1).
- The discriminability index is a signal-to-noise ratio related to the amount of overlap between the normal and abnormal parameter distributions. It is affected by the characteristics of the parameter being measured and the technology used to measure it, and it determines the proportion of true alarms to false alarms for a given β.
- As the decision criteria β is made more restrictive, the number of false alarms decreases, but the number of misses increases.
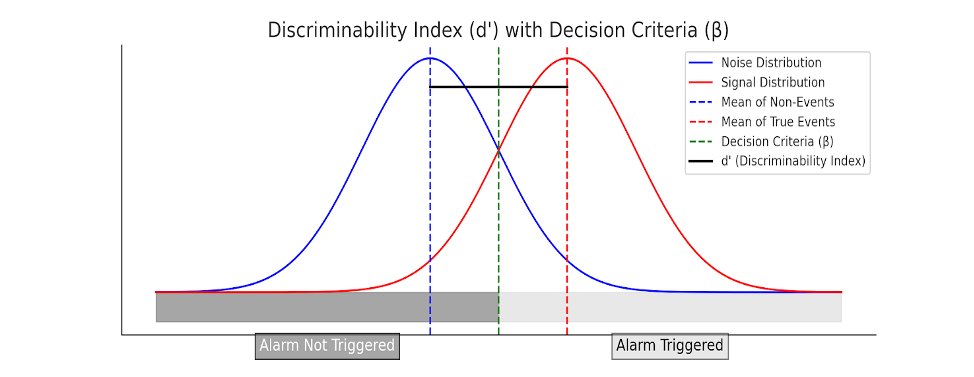
Figure 1. Alarm categories relative to true events and nonevents (random noise), both of which are distributed around an average parameter value. These two distributions often overlap. Alarm effectiveness depends both on the decision criteria/threshold (β) and discriminability index (d’).
Sensitivity and Specificity
- The sensitivity and specificity of a monitoring system can be derived from four alarm categories of a hit, correct rejection, false alarm, or miss:
- Sensitivity is the likelihood that when an event occurs, it will be detected (i.e., the “hit rate”). It is equal to the number of true alarms (“hits”) divided by the total number of events (“hits” plus “misses”)

-
- Specificity is the likelihood that when the situation is normal, no event will be indicated (i.e., correct rejection rate) and is equal to the number of correct rejections divided by the total number of normal cases (correct rejections plus false alarms).

- The positive predictive value of an alarm is the likelihood that when an alarm sounds, it indicates a true event or abnormality, i.e., the number of true “hits” divided by the total number of alarms (“hits” plus “false alarms”).

Types of Alarm Problems
- Xiao and Seagull1 define three categories of alarm problems:
- False alarms: no real danger exists, yet an alarm is triggered by interference, artifact, or overly conservative alarm thresholds.
- For example, electrocautery can produce electrocardiogram (ECG) artifacts on the vital sign monitor or a high airway pressure alarm on the ventilator in a context where higher peak pressures are expected and tolerated because plateau pressure remains acceptable.
- Nuisance alarms: indicate state changes that are not dangerous in the context in which they are set off
- For example, the vital sign monitor alarms about an abnormal arterial line tracing while an arterial blood gas sample is being drawn or the ventilator circuit triggering a disconnection alarm after being intentionally disconnected to reposition the patient.
- Inopportune alarms: an alarm occurs at the wrong time.
- For example, during a major disturbance, many alarms are sounding at once, causing the alarm to go unnoticed when it might otherwise have been of interest to the provider.
Alarm States, Modifications, and Fatigue
- Alarm states can be described using the following terms:4
- “Enabled/disabled” describes an alarm system as a whole, such that it will or will not detect events.
- “Sounding/not sounding” describes the activity of an individual annunciator (i.e., when an alarm is enabled and an event is detected, the alarm will sound. If the alarm is enabled and there is no event detected, it will not sound).
- “Alarm suppression” refers to when alarms are sounding, and the annunciator is silenced or briefly suspended. Alarm systems can allow permanent silencing of the auditory annunciators while leaving visual annunciators active.
- Alarm Modifications:4
- “Latched alarms,” once sounded, will continue to sound until silenced or “dismissed” by interaction with the monitor.
- “Unlatched alarms” will only sound if the criteria for sounding are met (i.e., the pulse oximeter will sound an alarm when saturations drop below the preset threshold and stop sounding when saturations return to normal).
- “Tiered” alarm systems prioritize the various alarms that may sound, with various levels of alarm annunciation.
- Alarm Fatigue
- Care providers can be exposed to hundreds of alarms per day, including false alarms. In one study on alarm density during elective cardiac surgery, the patient monitors and anesthesia workstation generated an average of 1.2 alarms per minute, 80% of which had no therapeutic consequence.3
- Physiologic monitors are designed with high sensitivity to avoid missing true events; however, this results in low specificity and low positive predictive value, with many false alarms.5
- Excessive alarms can result in sensory overload and desensitization, referred to as “alarm fatigue,” resulting in a lack of response by the provider.5
- Alarm fatigue contributes to adverse patient outcomes. The US Food and Drug Administration has reported over 500 alarm-related patient deaths in a 5-year period.2
Potential Solutions and Future of Alarms
- Alarm fatigue is a complex issue; however, several potential solutions have been proposed.
- Clinicians can modify alarm limits and threshold settings for each patient, such that they are appropriate for the clinical context, and disable nonessential alarms. This has been shown to decrease perceived workload and improve the accuracy of alarm response and overall satisfaction.6
- Changing alarm processing algorithms could potentially decrease the number of nuisance alarms and false alarms; for example, a short alarm delay for very minor threshold violations would prevent alarms caused by brief, inconsequential events.6
- Developing alarm algorithms that integrate multiple monitoring parameters (i.e., integrating ECG, pulse oximetry, and noninvasive blood pressure monitoring) may improve the positive predictive value of alarms and reduce the number of false alarms.2
- Developing alarms based on trends in physiologic parameters rather than individual data points may have better positive predictive value.5
- Reducing alarm volume, particularly for alarms that do not indicate a life-threatening condition, may help mitigate alarm fatigue.6
- Disposable monitors can be responsible for alarm artifacts, especially when repositioned. Ensuring proper skin preparation and routine replacement of ECG electrodes can decrease false alarms.5
References
- Xiao Y, Seagull FJ. An analysis of problems with auditory alarms: Defining the roles of alarms in process monitoring tasks. Proc Hum Factors Ergon Soc Annu Meet. 1999;43(3):256-60. Link
- Ruskin KJ, Hueske-Kraus D. Alarm fatigue: Impacts on patient safety. Curr Opin Anaesthesiol. 2015;28(6):685-90. PubMed
- Schmid F, Goepfert MS, Kuhnt D, et al. The wolf is crying in the operating room: Patient monitor and anesthesia workstation alarming patterns during cardiac surgery. Anesth Analg. 2011;112(1):78-83. PubMed
- Alarms in clinical anesthesia. Anesthesia Key. Published 2019. Accessed April 20, 2024. Link
- Cvach M. Monitor alarm fatigue: An integrative review. Biomed Instrum Technol. 2012;46(4):268-77. PubMed
- Ruskin KJ, Bliss JP. Alarm fatigue and patient safety. APSF Newsletter. 2019;34(1):1-28. Link
Copyright Information
This work is licensed under a Creative Commons Attribution-NonCommercial-NoDerivatives 4.0 International License.